
Man-made heat or, more precisely, anthropogenic heat flux (AHF), refers to the heat dissipated by human activities (e.g., through electricity generation, industry, transport). Several works have explored its possible effect on the Earth’s global climate system, some of them arguing that it could become a major cause of global warming (Chaisson 2008) or, at least, that it should be taken into account when allocating funding between competing energy technologies (Cowern & Ahn 2008). Indeed, as low-carbon energy sources, such as nuclear power, produce large amounts of heat, limiting global warming would be even harder than expected in the event heat has a significant impact. Recent works (Zhang & Caldeira 2015, Firth et al. 2019, Jin et al. 2019) have updated those first estimates. They have shown that the global effect of the AHF is currently negligible, especially when compared to the radiative forcing caused by greenhouse gas emissions. In this paper, we explore if this will remain valid in the future by computing the AHF for a couple of long-term energy projections.
The simplest derivation of the radiative forcing due to the AHF
The heat dissipated in the atmosphere by human activities, namely the AHF, modifies energy fluxes in the Earth’s climate system. It is therefore a potential driver of changes in the global average temperature. This can be quantified by computing the radiative forcing in units watts per square metre associated with the AHF.
The simplest derivation of the radiative forcing of the AHF can be obtained by dividing the annual world primary energy production from non-renewable sources by the surface of the Earth. By doing so, we assume that all the energy is eventually dissipated as heat, and we neglect transient effects due to energy storage. Renewables (wind, solar, hydro, geothermal and biomass powers) are excluded since they are converting solar energy that is already included in the Earth’s radiative budget. The non-energy use of oil, which presently represents about 5% of the total primary energy supply, is also excluded.
According to the data from the International Energy Agency, the total primary energy supply was 606 EJ in 2019. The share from non-renewable sources (excluding the non-energy use of oil) was 79.4%, i.e. 481 EJ. It corresponds to a radiative forcing of 0.030 W/m². This is in full agreement with previous estimates (Nordell 2003, Block et al. 2004, Flanner 2009, Chaisson 2008, Zhang & Caldeira 2015, Lu et al. 2017) and confirms that the radiative forcing due to AHF is negligible since it represents about one hundredth of the total anthropogenic radiative forcing of 2.72 [1.96 to 3.48] W/m² in 2019, as already pointed out by Covey et al. (2005) and Gumbel & Rodhe (2005).
Yet, AHF-caused forcing might become more and more important in the future if energy use increases. This was the reasoning of Chaisson (2008) when he concluded that AHF would become a major cause of global warming. Indeed, assuming that primary energy use will keep on growing at about 3% each year with an energy mix kept unchanged to what it currently is, the radiative forcing due to AHF will be ~0.1 W/m² by 2080 and ~1 W/m² by 2200. However, this line of argument collides with ongoing trends, notably the increasing share of renewable sources, gains in energy efficiency and more generally, the fact that most developed countries do not actually follow an ever-increasing energy demand. It is likely that this will moderate the rise of AHF compared to this first estimate, even if the implementation of carbon dioxide capture and storage (CCS) solutions, which may allow to reach carbon neutrality despite a continued use of fossil fuels (see e.g. the Shell’s Sky scenario), or the development of space-based solar power, would contribute to the AHF. A recent report for the UK government has indeed concluded that ‘space solar power is technically feasible, affordable, and could both bring substantial economic benefits for the UK, and could support Net Zero pathways’. Other countries, such as China, Japan and the US, have also shown interest in the concept. The report expects space-based solar power to deliver 15% of UK energy in 2040. This might be of concern notably because the AHF adds up to other forcing agents like greenhouse gases.
Detailed projections of the AHF based on two SSP-RCP scenarios
More detailed projections of the primary energy mix can be used to carefully assess the effect of the AHF in the future. The collection of socioeconomic pathways (SSPs) used in IPCC’s reports, for instance, provide alternative scenarios that feature different energy projections. They have recently been combined with greenhouse gas emission scenarios, or representative concentration pathways (RCPs), in the form of SSP-RCP scenarios.

In SSP4-3.4, which is of interest as an intermediate mitigation trajectory allowing the world to stabilise around +2°C above preindustrial levels, the total primary energy supply is expected to become flat after 2060 at about 800 EJ per year, with a decreasing share of fossil fuels (Fig. 1). The associated AHF radiative forcing would peak in 2040 at 0.036 W/m² and then drop below its present level (Fig. 2).
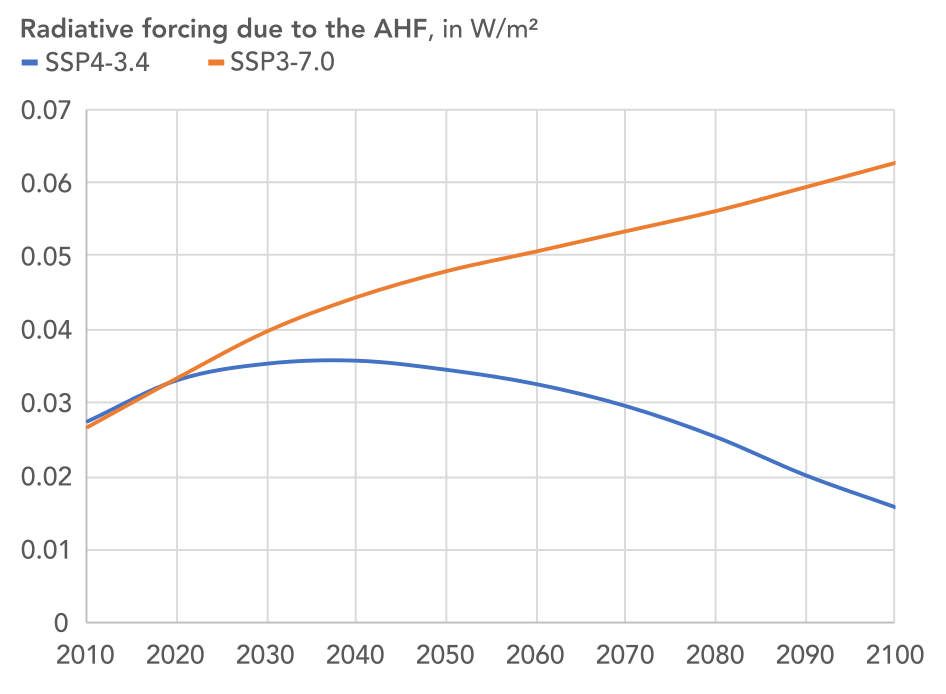
In SSP3-7.0, which represents an unmitigated baseline scenario leading to +4°C in 2100, and much more beyond, the total primary energy supply is expected to keep growing up to 1,200 EJ/yr in 2100 with an energy mix still largely based on fossil fuels (Fig. 1). The associated AHF radiative forcing increases as well, up to 0.06 W/m² in 2100, that is twice its present level (Fig. 2).
In both cases, the radiative forcing due to the AHF remains negligible compared to that of greenhouse gases. Indeed, for AHF to generate a radiative forcing of 1 W/m², the primary energy demand would have to reach at least ~16,000 EJ/yr. This is 27 times its present level. Assuming that the world population could stabilise around 10 billion people after 2100, this would imply a supply of ~1,600 GJ per capita. To date, the highest level of energy demand per capita in the world is met in Qatar, at ~750 GJ per capita, and the worldwide average is ~80 GJ per capita.
As a consequence, the AHF could become a significant forcing agent only if (i) the world energy mix is mainly based on non-renewable or extraterrestrial energy sources, such as fossil fuels, nuclear (fission or fusion, equivalently) or space-based solar power, and if (ii) new, extremely energy-intensive technologies emerge and become widely used, so that the demand per capita shoots up far beyond (20-fold) its present level, in spite of other natural limits, such as resource depletion and climate change.
Possible AHF evolutions in the far future
It must be noted that both conditions have to be met simultaneously, which seems very unlikely by 2100. This is in fact why the AHF forcing is not considered in most climate studies. However, before discarding this effect forevermore, it is worth investigating its evolution in the far future. To this end, we will extend the two SSP-RCP scenarios we have considered up to the year 2500. Of course, going beyond traditional end-points to projections is extremely speculative, since it is impossible to foresee major shifts in policies, population or technology, as well as any breakthroughs or collapses. The main idea here is to highlight the limits by simplistically extrapolating historical trends.
In SSP3-7.0, the economic growth rate becomes nearly constant after 2050, at 0.9% per year. In order to extend this projection in the far future for illustration purposes, we assume that (i) the world economic growth rate remains flat at 0.9 % per year from 2100 to 2500, and (ii) that the historic correlation between primary energy demand and economic growth holds in the future.
We choose to model this correlation using a power-law model, which supposes that the rate of energy intensity improvements depends on the economic growth rate, and therefore, that both become constant after 2050. Yet, diminishing returns may be expected and it is likely that energy intensity will not improve indefinitely, as gains are ultimately governed by physical limits as was shown by Ward et al. (2016). As a consequence, we present alternative scenarios where energy intensity cannot be improved further after a given year (Fig. 3). The earlier the saturation, the higher the energy demand in 2500.
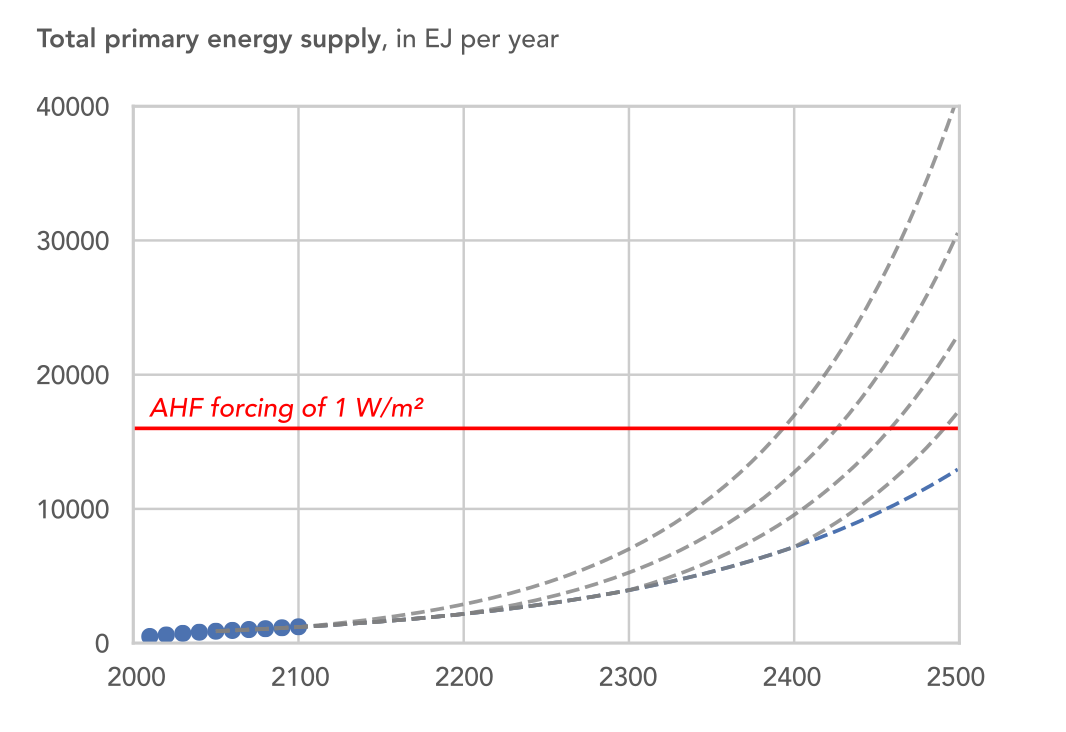
We find that AHF could become a significant forcing agent but likely not before several centuries (around 2400-2500). However, in these scenarios, energy demand reaches extreme values, always above 10,000 EJ/yr and possibly as high as 40,000 EJ/yr. A simple examination shows that burning all proven reserves of oil, gas and coal as of 2017 would release about 48,000 EJ, barely meeting the world demand for a single year. Burning all technically recoverable fossil fuel resources could generate 740,000 EJ, which could satisfy such a high global demand for only a couple of decades. Even if resource estimates are subject to huge uncertainties and may be significantly revised, they pose very serious limitations to these scenarios. Moreover, in SSP3-7.0, ongoing climate change due to greenhouse gases is left mostly unmitigated, and therefore associated damage will likely prevent mankind from reaching such GDP and energy demand levels. That is why it may be more relevant to study AHF in SSP4-3.4 than SSP3-7.0.

In SSP4-3.4, both GDP annual growth rate and energy intensity are projected to decay exponentially and eventually reach a plateau. The fact that energy intensity reductions due to substitution or efficiency cannot be maintained indefinitely is consistent with the findings of Ward et al. (2016). We extend these two projections to the year 2500 to derive a possible evolution of the world primary energy demand in SSP4-3.4 (Fig. 4). As energy intensity gains begin to stagnate, energy demand starts to rise again, reaching ~3,500 EJ/yr in 2500.
In such a scenario, the primary energy demand would induce an additional AHF radiative forcing of, at most, 0.1 W/m² in 2300, depending on the energy mix. It would be responsible for a global average temperature rise of about 0.1°C. This remains low compared to the global temperature rise due to greenhouse gases in this scenario (+1.8°C; Meinshausen et al. 2020), confirming that the AHF will most likely continue to play a very limited role in the Earth’s climate system.
The AHF forcing yet plays a role at the local scale
The AHF radiative forcing is far smaller than other forcing agents like greenhouse gases notably because it is averaged by the whole Earth’s surface. At the local scale however, the release of heat into a limited area, such as a city, can have a much more important effect. It typically ranges from 20 to 70 W/m² (Crutzen 2004), with far more extreme values reported locally, for example over 400 W/m² in daytime in central Tokyo (Ichinose et al. 1999).
This phenomenon is known as the urban heat island effect (UHI). It has been extensively documented, with detailed studies available for more than a hundred cities and regions (Santamouris 2015). It is complex since its magnitude depends on multiple factors, notably the physical, structural and morphological characteristics of the area considered (Oke et al. 1991). In his review, Santamouris (2015) reports that the associated temperature increase varies between 0.4°C and 11°C in 101 Asian and Australian cities and regions, with average value and standard deviation of 4.1°C and 2.3°C, respectively.
In the summer period, the UHI effect can have severe consequences on both human health and welfare, mainly through heat exposure and associated air pollution. It notably exacerbates the effects of heat waves in cities (e.g. Heaviside et al. 2017), such as the one that caused about 70,000 deaths in Europe in 2003 (Robine et al. 2008). It also impacts the energy consumption of buildings by increasing the demand for cooling and diminishing the efficiency of air-conditioners — whose use, moreover, causes further urban warming (Takane et al. 2019). It is estimated that the UHI increases electricity demand by a few percent (0.5-8.5%) with every 1°C increase (Santamouris et al. 2015).
Conclusion
When considered globally, the effect of the AHF is to modify natural energy fluxes by adding excess heat from non-renewable sources. Its effect on the Earth’s climate is real, but far smaller than other forcing agents like greenhouse gases.
For AHF to have a significant impact, it would be necessary that the world primary energy demand increases by more than one order of magnitude and that the energy mix remains largely non-renewable. This seems unlikely in the forthcoming centuries since a soaring non-renewable energy use will imply unmitigated climate change, which would be a far more pressing concern than the AHF, and would ultimately face resource limitations. In the far future however, the development of nuclear fusion and space-based solar power could have the ability to generate significant amounts of heat, so that the Earth’s climate system could eventually be affected, provided that the product of the world population and the energy use per capita becomes large enough.
At the local scale, on the contrary, the urban heat island is already a concern. As it is due to localised heat released by energy consumption regardless of its origin, it will not be limited by the development of renewable sources. While climate change makes heat waves more frequent, severe and long-lasting, their effect is exacerbated in urban areas due to the UHI effect (Founda & Santamouris 2017). This, as well as intensifying urbanization, implies an increased thermal risk for mankind in the future.
D. Chuard thanks Sophie Parison for providing him with insightful references and comments on the urban heat island effect.